Downscaling
The Earth’s climate is always changing, by virtue of various reasons that can be attributed to internal variability, natural forcings and human-caused forcings, where, internal variability is the change in climate without forcing at all, natural forcings are changes caused due to natural phenomena such as volcanic eruptions and human-caused forcings are variabilities due to various anthropogenic activities (NOAA, 2014). The effects of climate change are interrelated with each other and impact the life on earth, putting every single species at risk. Thus, it is crucial to understand the changes in climate and predict future scenarios.
General Circulation Models or Global Climate Models (GCMs) are models that simulate the present and future atmospheric scenarios which are used for forecasting weather, understanding the climate and for predicting the future climate change. GCMs work at the continental and hemispheric spatial scale (>104 km2) concerning the abstrusity and the magnitude of the global system and its accuracy decreases at progressively finer spatial scales (Ghosh & Mujumdar, 2008). Thus, GCMs are of little help when it comes to the representation of local sub-grid scale features and dynamics (Xu, 1999), which are of much importance pertaining to climate change impact assessment studies. GCM simulates the atmosphere, oceans, land surface, sea ice, etc, which are studied at the global scale, whereas hydrological models simulate precipitation, temperature, streamflow, evapotranspiration, etc, which varies largely at small scales. Figure 1 illustrates the working of a GCM grid and the physical processes involved within a grid.
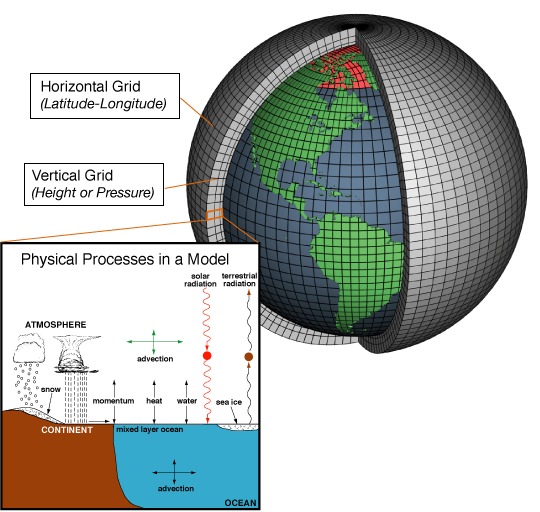
Figure 1: Operation of a GCM grid and the physical processes involved (Source: NOAA 2017 (http://climateilluminated.com/GCM_models/slides/1_GCM_models.html))
Hydrological impacts of climate change:
As hydrologists, we are interested in the impacts of climate change on the hydrological aspects. The variability in climate has been observed as precipitation events, heat waves and droughts with greater intensity and variability over the past few decades (Laflamme et al., 2015). Precipitation extremes are largely responsible for the climate-related damages caused, making them the main concern. Also, shifts in ecosystem distributions, changes in morphology and behaviour of species, trigger in the extinction of species, has also been observed due to the changes in natural systems, caused by extreme precipitation events. Apart from this, extreme precipitation causes flooding leading property and economic losses, crop damage, structural damage and breakdown of public health service (Laflamme et al., 2015). Hence, the prediction of extreme events and their consequences becomes extremely important considering the socio-economic scenarios. Figure 2 illustrates the impact of climate change on hydrological models.
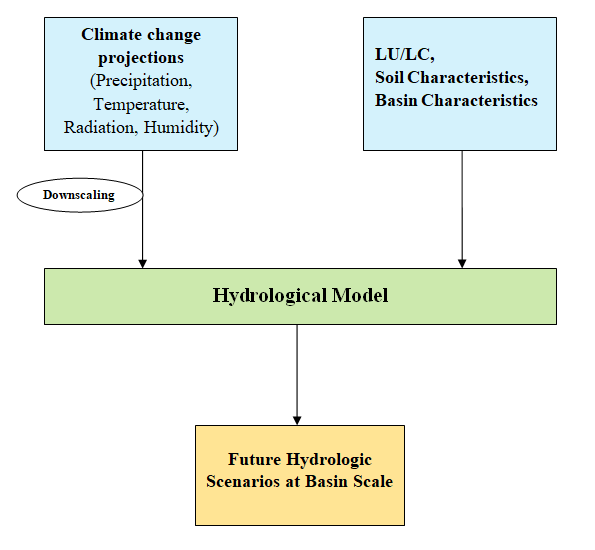
Figure 2: Impact of climate change on Hydrological models
The outputs from the GCM cannot be used directly as inputs in hydrological models considering the variation of spatial scale at which the models work. Table 1 shows the efficiency of GCMs for simulations on a different scale. As the grid size decreases, the ability of GCM decreases whereas the parameters that are not well simulated by GCM at smaller grid scales such as evapotranspiration runoff, soil moisture, etc are of greater importance in hydrological modelling.
In order to bridge this gap between the General Circulation Models and regional or local hydrological models, techniques have been developed, inherently known as Downscaling techniques. Downscaling techniques are approaches used to relate large scale climate variables to regional or local scale hydrologic variables, which are to be modelled in climate change impact assessment studies (Ghosh & Mujumdar, 2008). Downscaling techniques are broadly classified as dynamic downscaling, statistical downscaling and change factor method, in which dynamic downscaling uses complex algorithms at a fine grid-scale, i.e., typically of the order 50km * 50km. Dynamical downscaling is computationally intensive and involves lateral boundary conditions and parameters that represent land-sea contrast, vegetation cover, etc to produce a Regional Climate Model (RCM) from a GCM. Change factor Method is used when RCM outputs are unattainable for sensitivity studies or if a quick evaluation of various climate change scenarios is imperative (Fowler et al., 2007).
Table 1: Gaps between GCMs ability and hydrology need (Xu, 1999)
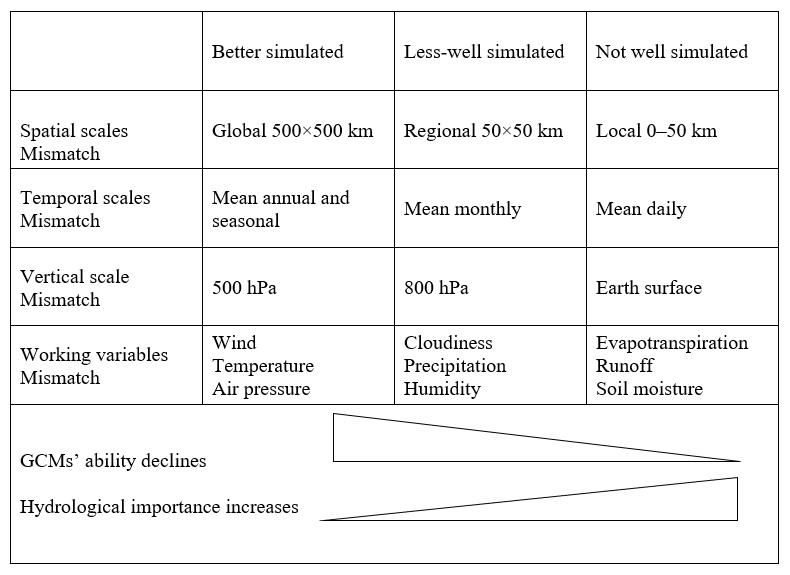
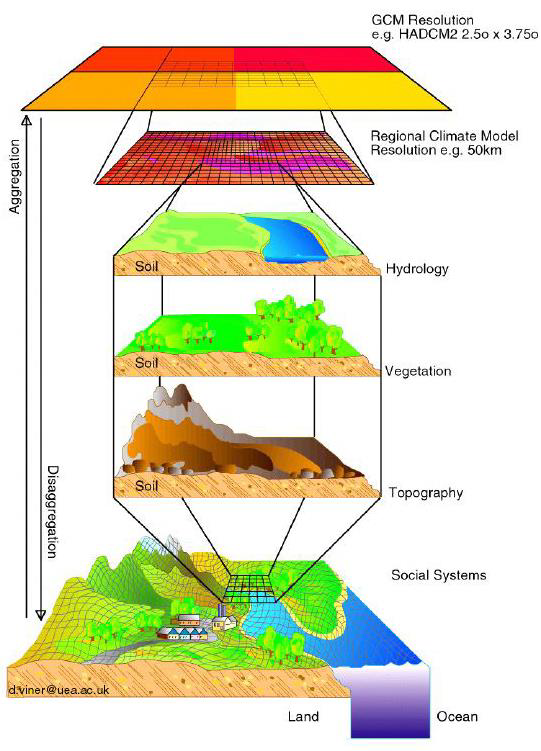
Figure 3: Graphical representation of downscaling (Source: https://www.researchgate.net/publication/267097515_A_Review_of_Downscaling_Methods_for_Climate_Change_Projections)
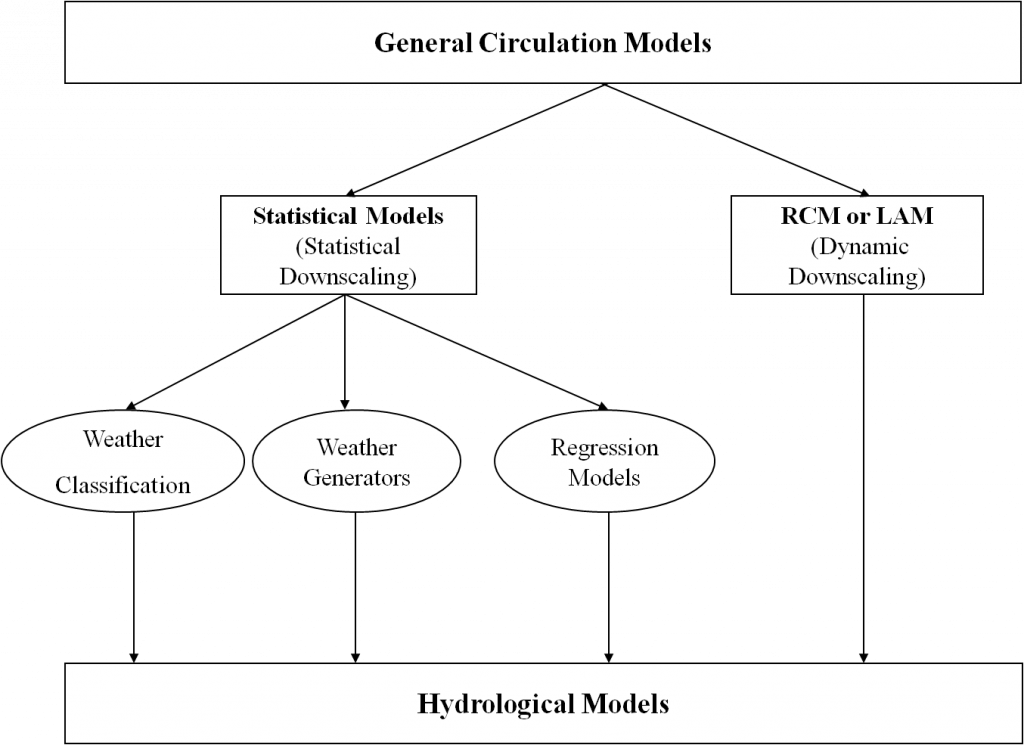
Figure 4: Downscaling Classification
References
Fowler, H. J., Blenkinsop, S., & Tebaldi, C. (2007). Linking climate change modelling to impacts studies: recent advances in downscaling techniques for hydrological modelling. International Journal of Climatology, 27(12), 1547–1578. https://doi.org/10.1002/joc.1556
Ghosh, S., & Mujumdar, P. P. (2008). Statistical downscaling of GCM simulations to streamflow using relevance vector machine. Advances in Water Resources, 31(1), 132–146. https://doi.org/10.1016/j.advwatres.2007.07.005
Laflamme, E. M., Linder, E., & Pan, Y. (2015). Statistical downscaling of regional climate model output to achieve projections of precipitation extremes. Weather and Climate Extremes, 12, 15–23. https://doi.org/10.1016/j.wace.2015.12.001
NOAA. (2014). Frequently Asked Questions (FAQ) on Global Warming, 1–17.
Xu, C.-Y. (1999). Climate Change and Hydrologic Models : A Review of Existing Gaps and Recent Research Developments. Water Resources Management, 13(5), 369–382. https://doi.org/10.1023/A:1008190900459
Xu, C. (1999). From GCMs to river flow: a review of downscaling methods and hydrologic modelling apporoaches. Progress in Physical Geography, 23(2), 229–249.