Climate Change Impact Assessment
There is wide scientific consensus over the three evident signals of climate change: i) increasing trend of average global temperature, ii) changes in circulation patterns affecting regional precipitation patterns, iii) rise in sea levels. These signals interfere with the regional hydrologic cycle impacting local water resources in terms of water availability (Ghosh and Mujumdar, 2008; Meenu et al., 2013), water quality (Rehana and Mujumdar , 2012), agricultural consumption of water (Rehana and Mujumdar , 2013), groundwater recharge and changed severity and frequency of extreme events such as floods and droughts. These climate change implications coupled with the rapid urbanization and industrial growth could have the potential to disrupt normal human life by depriving them of water, food, and livelihood security. Therefore to design and implement climate adaptation policies, it is important to understand its impact at local levels. The following sections highlight the process involved in the Impact Assessment Study, and challenges in making adaptation decisions.
Impact Assessment and adaptation
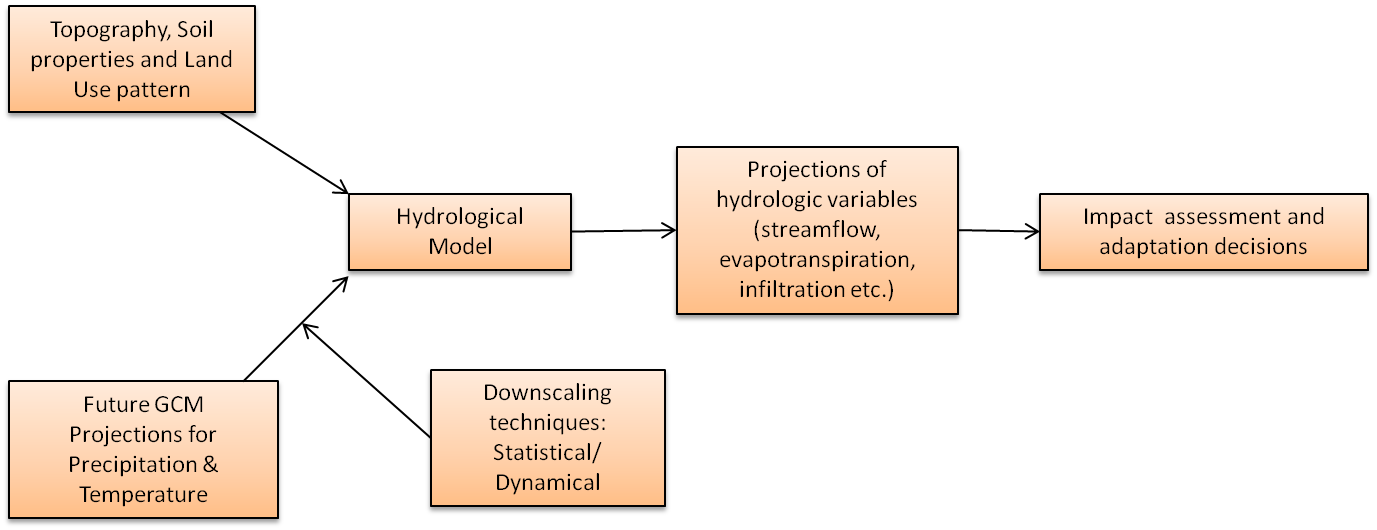
Figure 1. Steps involved in Climate Change Impact Assessment and Adaptation (Mujumdar, 2011).
Although, a GCM helps us to understand how climate functions and how it can be influenced by various forcings, but it is considered to be more uncertain while simulating hydrological variables of interest in climate change impact studies. Because, the performance of GCMs reduces while simulating hydrologic variables such as rainfall, streamflow and evaporation, compared to the performance while simulating climate variables (Wilby et al., 1998). These hydrological variables of interest could be precipitation at an urban scale, flow in a stream, evapotranspiration from crops and vegetation, groundwater recharge etc. Therefore, to design climate change adaptation solutions, we employ Hydrologic models. At the catchment scale, hydrologic models simulate various physical, chemical and biological processes that are concerned with the water, such as catchment runoff, infiltration, in-stream water quality, sediment transport, growth of microbes in water bodies etc. Physically-based hydrologic models require inputs on catchment characteristics (such as topography, soil properties and land use patterns) along with the hydroclimatic variables such as precipitation, temperature, relative humidity, solar radiation etc. These models are first calibrated and validated based on the historical observed data, and then are fed with the future projections of hydroclimatic data to assess the impact of climate change on local water resources.
GCMs, provide us the projections of various hydroclimatic variables under future greenhouse gas emission scenarios. These projections are available at spatial scales that are too coarse for the effective use of hydrological models at local scales. Therefore, to address this scale miss-match various techniques (Statistical/ Dynamical downscaling) are being employed to downscale GCM outputs at the local scale. The statistical downscaling approach establishes an empirical relationship between GCM-simulated climate variables (predictors) and regional-scale hydrological variables (predictands). An underlying assumption in this method is the stationarity of the developed relationship under changing climate. Dynamical downscaling involves the high-resolution regional climate models (RCMs), that are nested within the GCM, operating at a sub-grid scale over a particular region of interest. The hydrologic model runs with this downscaled GCM data gives the projections of a hydrologic variable of interest such as future streamflow. These projections can facilitate the estimate of likely changes into these variables in comparison with the historical observations.
The procedure described in Figure 1 is subject to the uncertainty resulting from the accuracy of input data, selection of a hydrological model and GCM, limitations of downscaling techniques, inaccuracy of historical datasets that are used in model calibration and validation etc. For sustainable management of water resources and adaptation planning, results from these impact studies must be conveyed to the policymakers in a way that is easy to grasp while highlighting the uncertainties associated with these results (Mondal and Mujumdar, 2014).
Variation in streamflow under changing climate will alter the flow duration curves, which is considered as one of the catchment signatures. Flow duration curve explains the exceedance probability associated with various streamflow values and is used while designing various structures such as dams, bridges, culverts etc. In hydropower projects, it is important to ensure the reliable generation of hydropower under the climate change scenario in order to meet consumer demand. A dam engineer has to optimize in between various demands such as hydropower generation, flood control, irrigation and. environmental flows while preparing reservoir operating policies. Raje and Mujumdar (2010) demonstrated an example of adaptive reservoir policies for hydropower generation from the Hirakud dam located in the Mahanadi river basin, India. Using the altered flow duration curves, they suggest the marginal reduction in the allocation for irrigation and flood control, for reliable hydropower generation under changing climate.
Impact Assessment and Adaptation in Urban setup (Mujumdar, 2011):
Changes in precipitation patterns caused by climate change would adversely impact the surface water and groundwater sources supporting the urban water infrastructure. Rising average temperatures exacerbated by the urban heat island effect and ever-increasing urban population would add stresses to the urban water availability due to increased demand. Unplanned urban growth, changes in the occurrence of extreme rainfall event along with the inadequacy of age-old stormwater infrastructure has worsened the existing issue of urban floods. Stormwater drains, pumping systems, detention tanks are part of an infrastructure that safeguards the city’s population from floods. The capacity of these components is determined based on the design rainfall intensities. These intensities at a location are obtained from the intensity-duration-frequency (IDF) relationships that are derived from the historical observations of rainfall and statistical analysis of maximum rainfall intensity. Under changing climate, it is expected to experience high-intensity rainfalls more frequently, altering the present IDF relationships. This will question the adequacy of existing drainage infrastructure, as they are expected to carry more discharge due to changed IDF relationships. This will compel local authorities to expand drainage capacity based on the revised hydrologic designs. Therefore, it becomes imperative for town planners to account for the likely changes in precipitation and temperature into their local climate change adaptation policies.
REFERENCES:
Ghosh, S., and Mujumdar, P.P. Statistical downscaling of GCM simulations to streamflow using relevance vector machine, Advances in Water Resources. (2008). 31, 132–146.
Meenu, R., Rehana, S., and Mujumdar, P.P. Assessment of hydrologic impacts of climate change in Tunga-Bhadra River basin, India with HEC-HMS and SDSM. Hydrological Processes. (2013). 27(11), 1572–1589.
Mondal, A., and Mujumdar, P.P. ‘Regional hydrological impacts of climate change: implications for water management in India’, Hydrological Sciences and Water Security: Past, Present, and Future (Proceedings of the 11th Kovacs Colloquium, Paris, France, June 2014), IAHS Publ. 365, 2015, pp 34-43. (DOI:10.5194/piahs-366-34-2015).
Mujumdar, P. P. ‘Implications of Climate Change for Water Resources Management’, India Infrastructure Report. (2011). Chapter-2, pp. 18-28.
Raje, D., and Mujumdar, P.P. Reservoir performance under uncertainty in hydrologic impacts of climate change. Advances in Water Resources. (2010). 33(3), 312–326.
Rehana, S., and Mujumdar, P.P. Climate change-induced risk in water quality control problems. Journal of Hydrology. (2012). 444-445, 63–77.
Rehana, S., and Mujumdar, P.P. Regional impacts of climate change on irrigation water demands. Hydrological Processes. (2013). 27(20), 2918–2933.
Wilby, R.L., Hassan, H., and Hanaki, K. Statistical downscaling of hydrometeorological variables using general circulation model output. Journal of Hydrology. (1998). 205(1), 1–19.